The obesity epidemic brought sharp focus onto population diets in Western industrialised countries, with recent emphasis on extrinsic, added sugars in particular. Extrinsic sugars do contribute to the development of overweight and obesity, insofar as they drive an energy surplus together with other energy-dense dietary constituents [1]. While the crux of the issue is increased overall caloric consumption in the diet, the evidence suggests that under free-living conditions, the primary issue with extrinsic sugars is that they are added to the diet without any compensatory reduction in energy intake [2]. Increasing the proportion of sugar in the diet under ad libitum conditions drives increased adiposity where calories are not reduced from other sources [2].
This has generated interest in the use of non-nutritive sweeteners [NNS], which encompasses both synthetic artificial sweeteners [AS] and non-caloric sweeteners of natural origin, as a strategy to reduce calorie intake in the population [3]. However, despite extensive toxicology studies, pre and post-market research, significant concerns continue to be raised in relation to the safety and efficacy of artificial sweeteners [4]. The most common concerns articulated relate to potential carcinogenic effects, contributions to weight gain, stimulation of blood glucose, activation of brain reward circuitry and stimulation of hunger or appetite [5].
For the purposes of this article, we’ll focus on AS only, specifically looking at acesulfame-K, aspartame, saccharin, and sucralose, which are the main AS in use [5]. This group of compounds fall under the umbrella of AS, as they can all be defined as having a greater sweetness intensity than sugar, allowing low levels to be added to foods and drinks as a caloric substitute [6]. However, the individual compounds all vary in sweetness potency, duration of sweetness, aftertaste, and mouth feel due to their structural differences, and have different pharmacokinetic profiles which warrant consideration of their health effects individually, and not under the umbrella term ‘AS’ [6].
Regulatory Processes and Approval for Use
This is an important first step in separating fact from fiction and fear in relation to AS: understanding the regulatory process and how the Acceptable Daily Intake [ADI] for any food additive is set. Compounds are not simply unleashed into the food environment for human consumption without regulatory authorities conducting safety evaluations, contrary to what many conspiracy theorists would like to believe. The AS listed above are approved for use in the U.S. by the Food and Drug Administration [FDA] and in the European Union [EU] through the European Food Standards Agency [EFSA], through a process involving submission of both scientific technical data and safety data [6][8].
Technical data relates to the chemical composition of the compound, source and manufacturing methods, stability of the compound across a range of food matrices, and sensory properties [6][8]. In addition, the full range of studies on safety must be contained in the submission process inclusive of the anticipate daily intake in the population, within different ages groups, from all dietary sources [6][8]. This safety information is derived from animal toxicology studies, which have specific directions for the type and design of studies required based on a system of “Concern Levels”: AS are considered a high concern level due to their potential high exposure in the population, and toxicity potential [6][8]. As a result of this concern level, studies must be conducted in animals with a similar pharmacokinetic profile to humans, and will assess both toxicity thresholds and sub-chronic toxicity for effects on reproduction, development, genotoxicity, carcinogenicity, and immunotoxicity [6].
Following animal toxicology studies, the “No Observable Adverse Effect Level” [NOAEL] is established, based on the lowest threshold of any toxicity observed in safety studies [7]. The ADI is then set by dividing the NOAEL by an uncertainty factor of 100 [7]. The technical data comes into play in assessing the potential health impact of the compound in the food supply, as exposure assessments combine data on the anticipated intake by reference to the concentrations of the compound in food/drinks together with the quantity of those foods/drinks consumed [7]. Like the establishment of the ADI, this is a very conservative assessment, particularly for children and the elderly, combining the maximum permitted level of the compound in foods together with the maximum level of consumption of food/drink [7].
The ADI & NOAEL vs. Consumption Levels
Putting this information into some context, the NOAEL for aspartame was set at 4g/kg/bw/day from long-term animal toxicology studies [7][8]. The ADI was then set at 50mg/kg in the U.S., or the equivalent of a 60kg adult consuming 4L of artificially sweetened soft drinks containing the maximum level of aspartame permitted under regulation [7]. Of course, no one food or drink contains the maximum permitted level of an AS; in the case of aspartame, average estimated intake is 1/10 the ADI [4mg/kg] while the highest quintiles of consumption still don’t exceed 30% of the ADI, with the 90th percentile consuming an average of 10mg/kg/d [9][10] Note that we are assessing consumption by reference to the ADI, not the NOAEL: even assuming an adult did consume the ADI for aspartame, that dose would still be 1/100 of the NOAEL, the lowest dose without any effect in long-term animal toxicology studies [7].
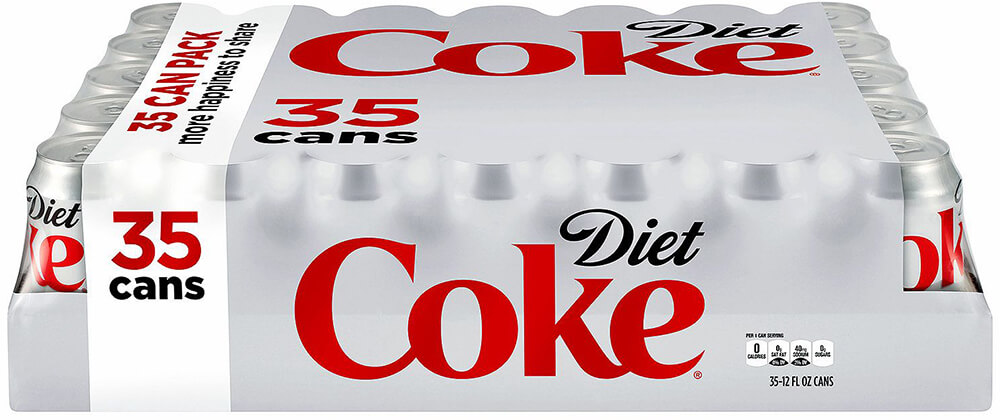
For saccharin, a common sweetener found in sweetener packs under brand names like ‘Sweet-N-Low’, the ADI is 5mg/kg/bw/d: to reach the ADI you would have to drink 800 12floz cans of diet soda sweetened with saccharin [11]. For acesulfame-K, the ADI is 15mg/kg, and average consumption in the U.S is 20% of the ADI over the course of a lifetime [11]. Sucralose, commonly known as ‘Splenda’, has an ADI of 5mg/kg and is one of the most widely used AS, ubiquitous in food and drink products: there is no bioaccumulation of sucralose [9]. There is an important point to make in light of the significant gap between toxicity and consumption: just because something has toxic potential doesn’t mean it is. There is a lower toxicity threshold for vitamin A, copper, or selenium than AS. What many people are falling prey to is a differentiation made only on the basis of the nature fallacy: that because AS are “artificial”, they are inherently negative in their health effects.
The ADI and NOAEL are important when we consider the purported adverse effects of AS: for example, a recent study looking at neurotoxic effects of aspartame used doses of 500mg/kg and 1,000mg/kg in rats [12], or 2,400% greater than the ADI and thus of no relevance to either established safety thresholds or population levels of consumption. Nonetheless, concerns over AS for human consumption remain, so due diligence is warranted in assessing human health effects.
AS & Carcinogenicity
Of the AS approved for use in the U.S., saccharin, aspartame, and acesulfame-K have the most concerns over potential carcinogenicity. Concerns in relation to saccharin arose from animal toxicology studies in which bladder cancer developed in rats administered high doses, however, further research found that the carcinogenic mechanisms identified in rodents were not applicable in humans, and no associations between saccharin consumption and cancer in humans have been found in subsequent studies [11][13].
Research on aspartame is generally controversial due to the strong associations between study outcomes and funding source: industry funded studies all attest to safety, while 92% of independently funded studies report adverse health effects, particularly in relation to body weight, diabetes/glucose regulation [14]. These inconsistencies mandated the need for an unbiased meta-analysis of empirical evidence in relation to the aforementioned issues, and cancer. A 2015 meta-analysis of carcinogenic bioassay rodent studies concluded that there was no significant relationship between various experimental doses of aspartame and occurrence of malignant tumors [14]. This is consistent with human observational epidemiology [7].
It should be noted that the 2015 meta-analysis included in its analyses 3 studies from the same research group in Europe, all of which purported to show carcinogenicity in rats and mice [15][16][17]. The findings of the latest study have been rejected by EFSA on the basis that the tumors observed in mice were not mechanistically relevant to human risk [18]. In addition, the researchers misdiagnosed hyperplasia as malignant tumors, and violated OECD protocols for testing by administering aspartame during fetal development [7][18]. Unfortunately, these studies highlight unscientific assertions that run contrary to the fact that there is no strong evidence that aspartame is carcinogenic [19][20]. This should be interpreted in the context of recent re-analyses of aspartame by both the FDA and EFSA concluding that the current ADI is appropriate having regard to current levels of consumption and potential risk [18][5].
Acesulfame-K has been the subject of concern due to its approval by the FDA coming prior to the standardization of animal carcinogenic bioassays, leading to criticism that the studies assessing carcinogenicity are inadequate [21]. As a “second generation” AS introduced after aspartame and saccharin, and approved in the late 1980’s, there is a lack of human observational epidemiology in relation to acesulfame-K and cancer [19]. Toxicology research by the National Toxicology Program failed to find any carcinogenic effect in rodent models [22], however, these toxicology studies failed to conform to the 24-month duration recommended by the FDA in its toxicology study design guidelines [23]. In the United States, controversy over the use of acesulfame-K has continued as the NTP has rejected submissions to reevaluate its safety [23]. However, the EU has ongoing toxicology monitoring programs which continually review AS and other food additives. In 2000, the EU Scientific Committee on Food [SCF] reevaluated acesulfame-K, including consideration of the claims that the original toxicology studies were inadequate, and found claims for carcinogenicity were not substantiated [24]. In 2009, the EU mandated that all AS in use had to be reevaluated by the EFSA, which replaced the SCF, including updated toxicology and technical data [8]. Acesulfame-K was concluded to be safe, and in fact, its permitted use was extended to children up to 3-years of age [25]. Notwithstanding the controversy in the US, which in reality is a regulatory issue, the ongoing scientific review by independent regulatory bodies in the EU suggests acesulfame-K is not a safety concern at habitual levels of consumption, which remain a fraction of the established ADI [26][24].
Sucralose, known under the brand name ‘Splenda’, is perhaps one of the most comprehensively researched AS with a strong safety profile, including for consumption in pregnancy and in children [11]. A recent review of toxicology studies found no evidence of carcinogenicity in long-term animal models or in human studies [27]. The research on sucralose is indicative of an important point to consider: toxicology programs are designed to elicit a response, and there is substantial regulatory oversight in ensuring rigorous standardization of the bioassay models [27]. To date, numerous international agencies and scientists in the field continue to support the safety of these compounds [5].
Often, the criticism to the lack of any associations in carcinogenic studies will be dismissed by cynical comments such as “who’s regulating the regulators”. I would point out that the EU has to date banned 1,300 compounds from use in cosmetics and personal care products, based on even preliminary evidence of toxicology [28]. The cynicism fails to stand up to an objective, unbiased assessment of the regulatory framework in which the safety of AS is assessed. That said, animal bioassays informing long-term human consumption health risks always carry a margin of uncertainty. In this regard, it is difficult from a scientific standpoint to consider the carcinogenic potential of AS a closed case. However, within current toxicology programs and at average consumption levels in the population, the current evidence does not support a carcinogenic effect of authorized AS in humans.
AS & Weight Regulation
There is a clear delineation between observational research and controlled trials. Several prospective human studies have observed increasing adiposity and cardiometabolic risk factors with high and/or regular diet soda consumption [29]. However, it is important to clarify that the increase in cardiometabolic risk factors is a consequence of increasing adiposity [29]. The relevant research question is the relationship between non-calorically sweetened beverage consumption and weight gain. Closer scrutiny of the observational research reveals that higher adiposity is associated with high levels of AS use, not the other way around [3]. This is consistent with the reverse causality observed between AS and body weight: those with high AS consumption are more likely to have a poor, high calorie diet and be overweight/obese [3].
There are multiple controlled trials looking at this issue. A recent meta-analysis examined 15 RCTs, finding that use of AS was clearly associated with lower body weight, BMI and waist circumference [30]. The included RCTs amounted to a large sample size, including 4 studies in children, and no single study found that AS use led to negative weight outcomes [30]. This is consistent with the expected purpose of AS substituting for sugar and/or calories in beverages and food products: to reduce total energy intake and facilitate weight loss, which is consistently observed in RCTs [3][30].
This does assume that compensations are not made in energy intake with substituting AS and increasing calories from other sources – the reverse causality observed in observational research. However, the observed reverse causality is a behavioral issue, and cannot be attributed to AS specifically. Controlled data supports basic nutrition fundamentals regarding energy intake, and when AS products substitute for calories, individuals lose weight [3][30]. However, certain literature has in fact suggested mechanisms by which AS may contribute to weight gain, including stimulating blood glucose and “tricking” the brain through activation of brain-reward circuitry which responds to sugar/sweet taste, stimulating appetite [31][32]. These concerns warrant a closer look.
AS & Blood Glucose Regulation
The main hypothesis here is that the sweet perception alters glycemic and insulin responses, and sweet receptors in the gut are activated in response to AS, increasing glucose uptake. In relation to the former, a trial in morbidly obese subjects but with normal insulin sensitivity [assessed by HOMA-IR] found greater peak plasma glucose levels and insulin response to a 75g oral glucose tolerance test [OGTT] 10-mins after a pre-load with 48mg of the AS sucralose [33]. This study has challenged prior research looking at sucralose, which was considered to have no impact on carbohydrate metabolism in multiple studies looking at glycemic responses [34].
It is difficult to reconcile the current literature in relation to glycemic or insulin responses from sucralose. In the SCF review of sucralose safety, a 6-month study in Type-2 diabetics found a consistent increase above baseline in HbA1c, a marker of long-term glycemic control, in subjects given 667mg sucralose daily. However, this occurred in the absence of impacts on any other markers of glucose regulation, including insulin or blood glucose levels [35]. The SCF review ultimately concluded that as the amounts administered were greater than consumption levels even in high consumers, any effect would be so small as to be clinically insignificant [35]. In the trial finding greater peak plasma glucose and insulin responses to an OGTT, the responses still remained within normal range for OGTT and amounted to nominal differences between sucralose group and controls [36]. The inconsistencies in the literature are synopsized by trials in sucralose: one study reported higher blood glucose levels, one study found lower blood glucose levels, and nine studies found no changes [36].
A recent systematic review of 28 trials of aspartame, sucralose, saccharin, and acesulfame-K confirmed the inconsistencies evident in the literature: some trials have found effects of AS on glucose metabolism while many have not found any interaction, and the significant heterogeneity between subjects, AS used, placebo, and outcome variables, limits comparisons [36]. Another issue is the comparison of AS to placebo, where arguably the appropriate trial design is a comparison with a caloric sweetener [3]. This is because, assuming AS do act to stimulate glucose uptake, this would occur in the context of low gut concentrations of glucose, while a caloric sweetener like sucrose would result in a greater amount of glucose absorbed [37]. This may explain why, in trials using OGTT, the observed differences in absorption are largely nominal [38].
Another issue is that the research in relation to the second limb of the hypothesis, the underlying mechanism of activating glucose transporters in the gut, is as inconsistent as the effects on blood glucose and/or insulin itself. One proposed mechanism is activation of glucagon-like peptide-1 [GLP-1], which has been assessed in 11 studies included in a systematic review: aspartame has been found to lower GLP-1, sucralose and acesulfame-K to increase GLP-1 concentrations [36]. Again, this is not consistent: a trial administering 72mg aspartame and 24mg sucralose or water before a 75g OGTT found no effect of either AS on GLP-1, insulin or glucose in Type-2 diabetics, although healthy subjects had a significantly higher GLP-1 area-under-the-curve from sucralose, but not aspartame [39].
An example of the noted limitations of trial design and resulting findings is evident in two trials. The first used diet soda as an intervention in healthy humans, with a beverage containing 46mg sucralose plus 26mg acesulfame-K, and found GLP-1 increased in response to OGTT compared to water [40]. However, the trial failed to control for compounds in the diet soda – including citric acid, potassium benzoate, phosphoric acid, potassium citrate, natural flavorings and colorings – which may have influenced the results [40]. Consequently, a subsequent trial administered 52mg sucralose, 200mg acesulfame-K, or 46mg sucralose plus 26mg acesulfame-K to healthy subjects, and found no effect of either treatment on GLP-1, blood glucose, or insulin concentrations (41). This is consistent with a trial showing no effect of sucralose administered through intraduodenal glucose feeding on GLP-1 or glucose uptake [42]. Taken as a whole, there are a number of trials that show some effect of AS on GLP-1 and glycemic response [39][40], while other studies [41][42] and a systematic review of 11 studies measuring GLP-1 [37] suggest that the majority of human studies have found no effect of AS on intestinal sweet taste receptors and glucose uptake. The biological basis for the limited studies finding such effect in humans remains unexplained [37].
A criticism of this area has been that, based on in vitro and animal model studies, many authors have reached outside the data to make speculative assumptions on the consequences of sweet-taste receptor activation on glucose regulation [37]. While potential mechanisms have been elucidated in vitro and in animal models, with limited supporting human data, the question begs: are the nominal effects of any clinical relevance? The overall body of evidence for a direct effect of AS on glycemic control is limited [3]. It would be an overreach on the basis of the evidence to say that AS influence blood glucose, insulin, and/or GLP-1 in humans; it would equally be an overreach to say they are biologically inert.
Activation of Brain Reward and Stimulation of Appetite
The hypothesis proffered in this regard is that the intense sweetness of AS activates responses in the brain regions controlling energy balance. The evidence suggests, however, that for hypothalamic responses to occur, there must be a pairing of sweet with energy content [11]. This is corroborated from sports nutrition research investigating the effects of carbohydrate mouth rinsing on performance, which suggested that the presence of glucose was necessary to elicit a central response [43]. To this end, a trial used functional magnetic resonance imaging [fMRI] to determine activation of brain-reward regions in response to either glucose, maltodextrin or saccharin intake [43]. Both the carbohydrate-containing solutions activated dopaminergic pathways which mediate reward-responses to food, areas which were unresponsive to saccharin [43]. This is consistent with a trial comparing sucrose to sucralose that found only sucrose led to any brain activation response [44]. These studies indicate that the presence of sweet alone is insufficient for activation of brain areas with a role in appetite or food-seeking behaviors, and such activation requires the coupling of energy content to sweet as would be found in simple sugars [43][44]
Notwithstanding studies which use fMRI to determine brain responses, arguments remain that AS diminish control over energy intake, and that the disconnect between sweetness and energy content precipitates further energy intake [45]. This does not appear to be borne out in the literature. In a systematic review and meta-analyses on the effect of AS consumption on energy intake, studies using pre-loads with AS beverages and subsequent ad libitum test meals found consumption of AS resulted in a reduction in overall energy intake, an effect consistent with longer-term RCT’s [45]. This is consistent with another systematic review which identified 7 studies investigating subjective appetite scores, none of which found any effect of AS on subjective appetite [36]. Taken as a whole, the literature does not appear to support any direct effect of AS on hunger and appetite [46], nor can it be said that AS “trick the brain” into a response in the absence of carbohydrate [43][44].
AS & The Gut Microbiome
Research on the human gut microbiome has demonstrated the influence on host health from the composition of gut bacteria, which is strongly impacted by diet [47]. To date, the primary driver of change in the human gut is the presence or absence of dietary fiber, which undergo selective fermentation by bacteria specializing in the degradation of indigestible plant matter [48]. Questions over the impact of AS on the gut microbiome have been generated largely from in vitro studies, with limited quality human evidence [49]. Recent evidence has found that alterations in the gut microbiome from saccharin, aspartame, and sucralose increased glucose intolerance in mice [50]. These results may not extrapolate to humans: aspartame is comprised of the amino acids phenylalanine and aspartic acid, and is broken down into its component parts in the human small intestine [7]. The conclusions of the study were based on interpretation of data from fecal samples extrapolated from rodents to humans, and the findings should be interpreted with caution [49].
An issue with this area of research is that established models and research designs have yet to be determined [49]. There is evidence that sugar alcohols commonly used as AS have been demonstrated to have a prebiotic effect, increasing concentrations of beneficial bacterial populations in humans [51]. Nonetheless, there remains suggestive mechanisms identified in animal models for metabolic consequences to altered microbiome composition as a result of AS intake [52]. The identified microbial compositions correlate with alterations observed in the microbiome in humans with metabolic disease [52]. These effects warrant further investigation in well-controlled human studies with recognized models. This area is, however, an example of the hyperbole surrounding AS: those against their use liberally cite the limited and questionable animal data as concrete evidence of an effect on the human microbiome, while those for their use casually assume a benign effect. The reality is at this moment in time, we cannot say definitively either way.
Conclusions
Can we say AS have a benign influence on human health? Can we say that there are no long-term consequences for human health from AS? In relation to both questions, the answer – which many people don’t want to hear – is “we’re not sure”. Overall, it does appear that much of the profoundly negative effects are exaggerated. Arguably, at current habitual levels of consumption in the population, AS would not appear to negatively impact human health to any identifiable degree: but that cannot be stated with authority having regard to grey areas in the literature and the reliance on animal models from toxicology to the microbiome.
Can we recommend AS beverages and foods as a means to improve health? AS can be an effective substitution for caloric-containing foods and beverages, provided those calories are not replaced elsewhere in the diet. Insofar as we are in the midst of an obesity epidemic, if AS use leads to a reduction in energy intake and facilitates weight loss, that must be considered a positive intervention. However, I do think health professionals should err on the side of caution with recommending overly liberal use. AS beverages and foods can be recommended in the context of overall nutritional best practices and should be used as a tool, not a crutch. In my opinion, that is a responsible position stand having regard for the literature.
References
- Khan, T. and Sievenpiper, J. (2016). Controversies about sugars: results from systematic reviews and meta-analyses on obesity, cardiometabolic disease and diabetes. European Journal of Nutrition, 55(S2), pp.25-43.
- Te Morenga, L., Mallard, S. and Mann, J. (2012). Dietary sugars and body weight: systematic review and meta-analyses of randomised controlled trials and cohort studies. BMJ, 346(jan15 3), pp.e7492-e7492.
- Gardner, C. (2014). Non-nutritive sweeteners. Current Opinion in Lipidology, 25(1), pp.80-84.
- Martyn, D., Nugent, A., McNulty, B., O’Reilly, E., Tlustos, C., Walton, J., Flynn, A. and Gibney, M. (2016). Dietary intake of four artificial sweeteners by Irish pre-school children. Food Additives & Contaminants: Part A, pp.1-11.
- Lohner, S., Toews, I. and Meerpohl, J. (2017). Health outcomes of non-nutritive sweeteners: analysis of the research landscape. Nutrition Journal, 16(1).
- Roberts, A. (2016). The safety and regulatory process for low calorie sweeteners in the United States. Physiology & Behavior, 164, pp.439-444.
- Marinovich, M., Galli, C., Bosetti, C., Gallus, S. and La Vecchia, C. (2013). Aspartame, low-calorie sweeteners and disease: Regulatory safety and epidemiological issues. Food and Chemical Toxicology, 60, pp.109-115.
- REGULATION (EC) No 1333/2008 OF THE EUROPEAN PARLIAMENT AND OF THE COUNCIL of 16 December 2008 on food additives.
- Butchko, H. (1997). Safety of aspartame. The Lancet, 349(9058), p.1105.
- Magnuson, B., Burdock, G., Doull, J., Kroes, R., Marsh, G., Pariza, M., Spencer, P., Waddell, W., Walker, R. and Williams, G. (2007). Aspartame: A Safety Evaluation Based on Current Use Levels, Regulations, and Toxicological and Epidemiological Studies. Critical Reviews in Toxicology, 37(8), pp.629-727.
- Shankar, P., Ahuja, S. and Sriram, K. (2013). Non-nutritive sweeteners: Review and update. Nutrition, 29(11-12), pp.1293-1299.
- Abhilash, M., Sauganth Paul, M., Varghese, M. and Nair, R. (2012). Long-term consumption of aspartame and brain antioxidant defense status. Drug and Chemical Toxicology, 36(2), pp.135-140.
- Gallus, S., Scotti, L., Negri, E., Talamini, R., Franceschi, S., Montella, M., Giacosa, A., Dal Maso, L. and La Vecchia, C. (2006). Artificial sweeteners and cancer risk in a network of case-control studies. Annals of Oncology, 18(1), pp.40-44.
- Mallikarjun, S. and Sieburth, R. (2013). Aspartame and Risk of Cancer: A Meta-analytic Review. Archives of Environmental & Occupational Health, 70(3), pp.133-141.
- Soffritti, M., Belpoggi, F., Esposti, D., Lambertini, L., Tibaldi, E. and Rigano, A. (2005). First Experimental Demonstration of the Multipotential Carcinogenic Effects of Aspartame Administered in the Feed to Sprague-Dawley Rats. Environmental Health Perspectives, 114(3), pp.379-385.
- Soffritti, M., Belpoggi, F., Tibaldi, E., Esposti, D. and Lauriola, M. (2007). Life-Span Exposure to Low Doses of Aspartame Beginning during Prenatal Life Increases Cancer Effects in Rats. Environmental Health Perspectives, 115(9), pp.1293-1297.
- Soffritti, M., Belpoggi, F., Manservigi, M., Tibaldi, E., Lauriola, M., Falcioni, L. and Bua, L. (2010). Aspartame administered in feed, beginning prenatally through life span, induces cancers of the liver and lung in male Swiss mice. American Journal of Industrial Medicine, 53(12), pp.1197-1206.
- Statement of EFSA on the scientific evaluation of two studies related to the safety of artificial sweeteners. (2011). EFSA Journal, 9(2), p.2089.
- Weihrauch, M. and Diehl, V. (2004). Artificial sweeteners—do they bear a carcinogenic risk?. Annals of Oncology, 15(10), pp.1460-1465.
- Bosetti, C., Gallus, S., Talamini, R., Montella, M., Franceschi, S., Negri, E. and La Vecchia, C. (2009). Artificial Sweeteners and the Risk of Gastric, Pancreatic, and Endometrial Cancers in Italy. Cancer Epidemiology Biomarkers & Prevention, 18(8), pp.2235-2238.
- Woods, D. (1996). US scientists challenge approval of sweetener. BMJ, 313(7054), pp.386-386.
- National Toxicology Program (2005). NTP toxicology studies of acesulfame potassium (CAS No. 55589-62-3) in genetically modified (FVB Tg.AC Hemizygous) mice and carcinogenicity studies of acesulfame potassium in genetically modified [B6.129-Trp53(tm1Brd) (N5) Haploinsufficient] mice (feed studies)mice. Natl Toxicol Program Genet Modif Model Rep., Oct(2), pp.1-113.
- Karstadt, M. (2006). Testing Needed for Acesulfame Potassium, an Artificial Sweetener. Environmental Health Perspectives, 114(9), pp.A516-A516.
- Mortensen, A. (2006). Sweeteners permitted in the European Union: safety aspects. Food & Nutrition Research, 50(3).
- European Food Safety Authority (2016). Safety of the proposed extension of use of acesulfame K (E 950) in foods for special medical purposes in young children. EFSA Journal, 14(4), p.4437.
- Lino, C., Costa, I., Pena, A., Ferreira, R. and Cardoso, S. (2008). Estimated intake of the sweeteners, acesulfame-K and aspartame, from soft drinks, soft drinks based on mineral waters and nectars for a group of Portuguese teenage students. Food Additives & Contaminants: Part A, 25(11), pp.1291-1296.
- Berry, C., Brusick, D., Cohen, S., Hardisty, J., Grotz, V. and Williams, G. (2016). Sucralose Non-Carcinogenicity: A Review of the Scientific and Regulatory Rationale. Nutrition and Cancer, 68(8), pp.1247-1261.
- Regulation (EC) No 1223/2009 of the European Parliament and of the Council of 30 November 2009 on cosmetic products.
- Fowler, S. (2016). Low-calorie sweetener use and energy balance: Results from experimental studies in animals, and large-scale prospective studies in humans. Physiology & Behavior, 164, pp.517-523.
- Miller, P. and Perez, V. (2014). Low-calorie sweeteners and body weight and composition: a meta-analysis of randomized controlled trials and prospective cohort studies. American Journal of Clinical Nutrition, 100(3), pp.765-777.
- Peters, J. and Beck, J. (2016). Low Calorie Sweetener (LCS) use and energy balance. Physiology & Behavior, 164, pp.524-528.
- Rogers, P. and Brunstrom, J. (2016). Appetite and energy balancing. Physiology & Behavior, 164, pp.465-471.
- Pepino, M., Tiemann, C., Patterson, B., Wice, B. and Klein, S. (2013). Sucralose Affects Glycemic and Hormonal Responses to an Oral Glucose Load. Diabetes Care, 36(9), pp.2530-2535.
- Brown, R. and Rother, K. (2012). Non-Nutritive Sweeteners and their Role in the Gastrointestinal Tract. The Journal of Clinical Endocrinology & Metabolism, 97(8), pp.2597-2605.
- Scientific Committee on Food of the European Commission. Opinion of the Scientific Committee on Food on sucralose. SCF/CS/ADDS/EDUL/190 Final 12/9/2000.
- Romo-Romo, A., Aguilar-Salinas, C., Brito-Córdova, G., Gómez Díaz, R., Valentín, D. and Almeda-Valdes, P. (2016). Effects of the Non-Nutritive Sweeteners on Glucose Metabolism and Appetite Regulating Hormones: Systematic Review of Observational Prospective Studies and Clinical Trials. PLoS One, 11(8), p.e0161264.
- Renwick, A. and Molinary, S. (2010). Sweet-taste receptors, low-energy sweeteners, glucose absorption and insulin release. British Journal of Nutrition, 104(10), pp.1415-1420.
- Grotz, V. and Jokinen, J. (2014). Comment on Pepino et al. Sucralose Affects Glycemic and Hormonal Responses to an Oral Glucose Load. Diabetes Care 2013;36:2530–2535. Diabetes Care, 37(6), pp.e148-e148.
- Temizkan, S., Deyneli, O., Yasar, M., Arpa, M., Gunes, M., Yazici, D., Sirikci, O., Haklar, G., Imeryuz, N. and Yavuz, D. (2014). Sucralose enhances GLP-1 release and lowers blood glucose in the presence of carbohydrate in healthy subjects but not in patients with type 2 diabetes. European Journal of Clinical Nutrition, 69(2), pp.162-166.
- Brown, R., Walter, M. and Rother, K. (2009). Ingestion of Diet Soda Before a Glucose Load Augments Glucagon-Like Peptide-1 Secretion. Diabetes Care, 32(12), pp.2184-2186.
- Steinert, R., Frey, F., Töpfer, A., Drewe, J. and Beglinger, C. (2011). Effects of carbohydrate sugars and artificial sweeteners on appetite and the secretion of gastrointestinal satiety peptides. British Journal of Nutrition, 105(09), pp.1320-1328.
- Ma, J., Chang, J., Checklin, H., Young, R., Jones, K., Horowitz, M. and Rayner, C. (2010). Effect of the artificial sweetener, sucralose, on small intestinal glucose absorption in healthy human subjects. British Journal of Nutrition, 104(06), pp.803-806.
- Chambers, E., Bridge, M. and Jones, D. (2009). Carbohydrate sensing in the human mouth: effects on exercise performance and brain activity. The Journal of Physiology, 587(8), pp.1779-1794.
- Frank, G., Oberndorfer, T., Simmons, A., Paulus, M., Fudge, J., Yang, T. and Kaye, W. (2008). Sucrose activates human taste pathways differently from artificial sweetener. NeuroImage, 39(4), pp.1559-1569.
- Rogers, P., Hogenkamp, P., de Graaf, C., Higgs, S., Lluch, A., Ness, A., Penfold, C., Perry, R., Putz, P., Yeomans, M. and Mela, D. (2015). Does low-energy sweetener consumption affect energy intake and body weight? A systematic review, including meta-analyses, of the evidence from human and animal studies. International Journal of Obesity, 40(3), pp.381-394.
- Raben, A. and Richelsen, B. (2012). Artificial sweeteners: a place in the field of functional foods? Focus on obesity and related metabolic disorders. Current Opinion in Clinical Nutrition and Metabolic Care, 15(6), pp.597-604.
- Graf, D., Di Cagno, R., Fåk, F., Flint, H., Nyman, M., Saarela, M. and Watzl, B. (2015). Contribution of diet to the composition of the human gut microbiota. Microbial Ecology in Health & Disease, 26(0).
- Walker, A., Ince, J., Duncan, S., Webster, L., Holtrop, G., Ze, X., Brown, D., Stares, M., Scott, P., Bergerat, A., Louis, P., McIntosh, F., Johnstone, A., Lobley, G., Parkhill, J. and Flint, H. (2010). Dominant and diet-responsive groups of bacteria within the human colonic microbiota. The ISME Journal, 5(2), pp.220-230.
- Daly, K., Darby, A. and Shirazi-Beechey, S. (2016). Low calorie sweeteners and gut microbiota. Physiology & Behavior, 164, pp.494-500.
- Suez, J., Korem, T., Zeevi, D., Zilberman-Schapira, G., Thaiss, C., Maza, O., Israeli, D., Zmora, N., Gilad, S., Weinberger, A., Kuperman, Y., Harmelin, A., Kolodkin-Gal, I., Shapiro, H., Halpern, Z., Segal, E. and Elinav, E. (2015). Artificial Sweeteners Induce Glucose Intolerance by Altering the Gut Microbiota. Obstetrical & Gynecological Survey, 70(1), pp.31-32.
- Beards, E., Tuohy, K. and Gibson, G. (2010). A human volunteer study to assess the impact of confectionery sweeteners on the gut microbiota composition. British Journal of Nutrition, 104(05), pp.701-708.
- Suez, J., Korem, T., Zilberman-Schapira, G., Segal, E. and Elinav, E. (2015). Non-caloric artificial sweeteners and the microbiome: findings and challenges. Gut Microbes, 6(2), pp.149-155.